Optimize thermogenic response -
Cypess, A. Identification and importance of brown adipose tissue in adult humans. New England Journal of Medicine , Anatomical localization, gene expression profiling and functional characterization of adult human neck brown fat.
Nature Medicine 19 , — Hanssen, M. Glucose uptake in human brown adipose tissue is impaired upon fasting-induced insulin resistance.
Diabetologia 58 , — Harms, M. Brown and beige fat: development, function and therapeutic potential. Nature Medicine 19 , Chu, D. Expression of adipocyte biomarkers in a primary cell culture models reflects preweaning adipobiology.
Journal of Biological Chemistry , Ramage, L. Glucocorticoids Acutely Increase Brown Adipose Tissue Activity in Humans, Revealing Species-Specific Differences in UCP-1 Regulation. Cell Metabolism 24 , — Jespersen et al. A Classical Brown Adipose Tissue mRNA Signature Partly Overlaps with Brite in the Supraclavicular Region of Adult Humans.
Cell Metabolism 17 , Hughes, D. Molecular evolution of UCP1 and the evolutionary history of mammalian non-shivering thermogenesis. Bmc Evolutionary Biology 9 , 4 Article PubMed PubMed Central Google Scholar. Di, F. Searching for classical brown fat in humans: Development of a novel human fetal brown stem cell model.
Stem Cells 34 , Seiler, S. Characterization of a primary brown adipocyte culture system derived from human fetal interscapular fat. Adipocyte 4 , — Zingaretti, M.
The presence of UCP1 demonstrates that metabolically active adipose tissue in the neck of adult humans truly represents brown adipose tissue. Faseb Journal Official Publication of the Federation of American Societies for Experimental Biology 23 , Kazantzis, M.
PAZ6 Cells Constitute a Representative Model for Human Brown Pre-Adipocytes. Frontiers in Endocrinology 3 , 13 Lee, P. Inducible brown adipogenesis of supraclavicular fat in adult humans.
Endocrinology , — Nishio, M. Production of functional classical brown adipocytes from human pluripotent stem cells using specific hemopoietin cocktail without gene transfer. Cell Metabolism 16 , — Morganstein, D. Human fetal mesenchymal stem cells differentiate into brown and white adipocytes: a role for ERRalpha in human UCP1 expression.
Cell Research 20 , — Javier, R. Cell Models and Their Application for Studying Adipogenic Differentiation in Relation to Obesity: A Review.
International Journal of Molecular Sciences 17 , Carobbio, S. Adipogenesis: new insights into brown adipose tissue differentiation. Journal of Molecular Endocrinology 51 , 75—85 Pisani, D. Frontiers in Endocrinology 2 , 87 Lidell, M. Evidence for two types of brown adipose tissue in humans.
Skurk, T. Primary Culture of Human Adipocyte Precursor Cells: Expansion and Differentiation. Humana Press, Li, Y.
Embo Reports 15 , Cigolini, M. Human brown adipose cells in culture. Experimental Cell Research , — Styner, M. Indomethacin Promotes Adipogenesis of Mesenchymal Stem Cells Through a Cyclooxygenase Independent Mechanism.
Journal of Cellular Biochemistry , — Lee, J. Triiodothyronine induces UCP-1 expression and mitochondrial biogenesis in human adipocytes. American Journal of Physiology Cell Physiology , C Lee, M. Optimal protocol for the differentiation and metabolic analysis of human adipose stromal cells.
Methods in Enzymology , 49 Shinoda, K. Genetic and functional characterization of clonally derived adult human brown adipocytes. Nature Medicine 21 , — Moisan, A. White-to-brown metabolic conversion of human adipocytes by JAK inhibition.
Nature Cell Biology 17 , 57—67 Xue, R. Clonal analyses and gene profiling identify genetic biomarkers of human brown and white preadipocyte thermogenic potential.
Xu, C. Direct effect of glucocorticoids on lipolysis in adipocytes. Molecular Endocrinology 23 , Wu, J. Beige adipocytes are a distinct type of thermogenic fat cell in mouse and human.
Cell , — Petrovic, N. Chronic peroxisome proliferator-activated receptor gamma PPARgamma activation of epididymally derived white adipocyte cultures reveals a population of thermogenically competent, UCP1-containing adipocytes molecularly distinct from classic brown adipocyte.
Klemm, D. Insulin-induced adipocyte differentiation. Activation of CREB rescues adipogenesis from the arrest caused by inhibition of prenylation. Journal of Biological Chemistry , — Senol-Cosar, O. Tenomodulin promotes human adipocyte differentiation and beneficial visceral adipose tissue expansion.
Nature Communications 7 , Price, N. Google Scholar. Kuri-Harcuch, W. Interruption of the adipose conversion of 3T3 cells by biotin deficiency: differentiation without triglyceride accumulation. Cell 14 , 53—59 A Modified Protocol to Maximize Differentiation of Human Preadipocytes and Improve Metabolic Phenotypes.
Obesity 20 , — Yeo, C. SGBS cells as a model of human adipocyte browning: A comprehensive comparative study with primary human white subcutaneous adipocytes. Scientific Reports 7 , Article ADS PubMed PubMed Central Google Scholar. Ouellet, V.
Brown adipose tissue oxidative metabolism contributes to energy expenditure during acute cold exposure in humans. Journal of Clinical Investigation , — Zilberfarb, V.
Human immortalized brown adipocytes express functional beta3-adrenoceptor coupled to lipolysis. Journal of Cell Science Pt 7 , — CAS PubMed Google Scholar.
Ahfeldt, T. Programming human pluripotent stem cells into white and brown adipocytes. Nature Cell Biology 14 , Nagasaki, H. Low-serum culture system improves the adipogenic ability of visceral adipose tissue-derived stromal cells. Cell Biology International 35 , Article PubMed Google Scholar.
Hauner, H. Cultures of human adipose precursor cells. Methods in Molecular Biology , Madsen, L. UCP1 Induction during Recruitment of Brown Adipocytes in White Adipose Tissue Is Dependent on Cyclooxygenase Activity. Plos One 5 , e Wang, M. The role of glucocorticoid action in the pathophysiology of the Metabolic Syndrome.
Kong, X. Glucocorticoids Transcriptionally Regulate miRb Expression Promoting Body Fat Accumulation Via Suppressing the Browning of White Adipose Tissue. Diabetes 64 , Barclay, J. Effects of glucocorticoids on human brown adipocytes.
Journal of Endocrinology , Jesus, L. The type 2 iodothyronine deiodinase is essential for adaptive thermogenesis in brown adipose tissue. Journal of Clinical Investigation , Asano, H.
Induction of Beige-Like Adipocytes in 3T3-L1 Cells. Journal of Veterinary Medical Science 76 , 57—64 Obregon, M. Thyroid hormone and adipocyte differentiation. Thyroid Official Journal of the American Thyroid Association 18 , Rajan, S.
Chronic hyperinsulinemia reduces insulin sensitivity and metabolic functions of brown adipocyte. Journal of Endocrinology , — Download references. Contract grant sponsor and numbers: The National Key Basic Research Program of China Grant No. Nanjing Maternity and Child Health Care Institute, The Affiliated Obstetrics and Gynecology Hospital of Nanjing Medical University, Nanjing, , China.
Nanjing Maternity and Child Health Care Institute, Nanjing Maternity and Child Health Care Hospital, Nanjing, , China. You can also search for this author in PubMed Google Scholar. XingYun Wang performed the experiments and wrote the paper.
LiangHui You interpreted the results of the experiments. XianWei Cui assisted in writing the manuscript. ChenBo Ji conceived and designed the experiments. XiRong Guo conceived the experiments, provided funding to regents, and approved the final version of the manuscript.
Juan Wen and PengFei Xu analyzed the data. The absence of CEBPα in mice prevents the development of WAT, but not BAT depots, indicating that lack of CEBPα can be compensated, probably by CEBPβ, during BAT development [ 41 ].
Furthermore, specific gene-regulatory networks govern the growth of BAT; the factors involved include PR domain-containing protein 16 PRDM16 [ 42 ] and peroxisome proliferator-activated receptor γ-coactivator-1α PGC1α [ 43 ]. PRDM16, but not PGC1α, specifically confers brown fat cell identity.
PRDM16 binds to and coregulates CEBPβ, PPARγ, PPARα, and PGC1α to promote brown fat-specific gene induction. PGC1α, which also coactivates PPARγ and PPARα, is involved in regulating mitochondrial biogenesis, oxidative metabolism, and thermogenesis.
Several studies have investigated the effects of different ligands with thermogenic potential in stimulating BAT activation [ 44 , 45 , 46 , 47 , 48 , 49 ]. CNS represents the main transducer of thermogenic responses, whose signals, including adrenergic stimuli and catecholamine [ 50 ], fuel sympathetic nerve activity SNA in BAT depots under control of specific environmental conditions e.
Other hormones, such as incretins and glucagon secreted by gut and pancreatic α-cells, respectively [ 51 , 52 , 53 , 54 ] appear to sustain BAT activity [ 29 , 30 , 55 ]. Table 1 summarizes current knowledge derived from in vitro, in vivo, and human studies regarding the emerging therapeutic effects of β3-adrenergic receptor agonists, incretin hormones, glucagon, their related pharmacological mimetics, and GLPbased multi-agonists, as new treatment options for enhancing thermogenesis and combat obesity.
In the last decade, β3-adrenergic receptor AR agonists have emerged as novel pharmacological approaches to counteract obesity and metabolic diseases. ARs are members of the family of G-coupled receptors [ 56 , 57 ] whose activation is induced by catecholamines [ 50 ]. The AR family is composed of three subclasses: α1-ARs, α2-ARs, and β-ARs [ 58 ].
Currently, three β-AR subtypes β1-AR, β2-AR, and β3-AR have been identified and are encoded by different genes. β3-ARs were identified in several tissues heart, blood vessels, gallbladder, gastrointestinal tract and their expression was found to be typically higher in brown depots [ 59 , 60 ], where non-shivering thermogenesis occurs in response to sympathetic nerve stimulation [ 27 , 28 ].
β3-ARs are known to exert metabolic effects by enhancing the rate of lipolysis [ 61 ] and release of long-chain non-esterified fatty acids LCFAs [ 62 , 63 ] via a Gs-cyclic adenosine monophosphate cAMP -protein kinase A PKA -dependent pathway Fig.
In this scenario, the activity of the thermogenic protein UCP1, which increases the conductance of the inner mitochondrial membrane to make mitochondria of BAT generate heat rather than ATP, is allowed by an LCFA-dependent mechanism [ 66 ]. Furthermore, LCFAs derived from β3-AR-dependent lipolysis act as an energy source to fuel thermogenesis in brown adipocytes [ 10 , 67 ].
Several agonists of β3-ARs have been developed, whose effects have been evaluated principally in vivo and in vitro, and to a lesser extent in human studies. Signalling pathways activated by drugs potentially affecting BAT activity and function: AMP-activated protein kinase AMPK , adrenoceptor beta 3 β3-AR , branched-chain amino acids BCAA , branched-chain keto acids BCKA , farnesoid X receptor FXR , gastric inhibitory polypeptide receptor GIPR , glucagon-like peptide 1 receptor GLP-1R , Glucagon G-coupled receptor GCGR , sirtuin-1 SIRT1 , peripheral nervous system PNS , peroxisome proliferator-activated receptor gamma coactivator 1-alpha PGC1α , phosphatidylinositol-3 kinase PI3K , PR domain-containing protein 16 PRDM16 , protein kinase A PKA , protein kinase B AKT , p38α mitogen-activated protein kinase p38 MAPK , ginsenoside Rb1 Rb1 , sympathetic nervous system SNP , exchange protein directly activated by cAMP EPAC , EPAC specific inhibitor ESI , CCAAT enhancer-binding protein α CEBPα , tricarboxylic acid TCA cycle, tumour necrosis factor receptor TNFR , tumour necrosis factor α TNF-α , thyroid receptor TR.
CL was the first adrenergic stimulator whose thermogenic and metabolic activity appears to occur by the activation of PKA—p38 mitogen-activated protein kinase MAPK pathway, as observed in mouse brown adipocytes Fig. In vitro studies have also clarified the underlying molecular mechanisms mediating the thermogenic response to CL, highlighting that this compound caused an increased mRNA expression of PGC1α [ 67 ], a gene involved in cold-induced thermogenesis and browning [ 68 ].
PGC1α requires deacetylation by sirtuin-1 SIRT1 Fig. The role of β3-adrenergic signalling in regulating thermogenic process was also established in 3T3-L1 adipocytes, in which the incubation with ginsenoside Rb1 Rb1 , a saponin derived from Panax ginseng , was shown to act as a β3-AR activator [ 71 ].
Rb1 upregulated SIRT1 together with the activation of downstream browning effectors, such as AMP-activated protein kinase α AMPKα , liver kinase B1, and acetyl-CoA carboxylase ACC , and increased the rate of lipolysis [ 71 ]. Moreover, Rb1 is also known to exert metabolic functions including suppression of triglyceride TG accumulation in vitro [ 72 ] via activation of AMPK [ 73 ], a key factor promoting energy production.
These studies suggest that Rb1 can decrease lipid accumulation by inducing the lipolysis—thermogenesis cascade [i. AMPKα, adipose triglyceride lipase ATGL , and UCP1] within WAT depots, confirming its therapeutic potential for counteracting obesity [ 71 ].
The thermogenic effect of the CL compound was also confirmed by in vivo studies. When infused in obese mice, this molecule increased EE and reduced body weight in association with increased UCP1-dependent thermogenesis in WAT [ 74 , 75 ].
Notably, CL requires mitochondrial fatty acid oxidation, since obese mice developed a resistance to CLinduced thermogenesis when carnitine palmitoyl transferase 2 CPT2 was genetically abrogated [ 74 ].
Furthermore, the efficacy of CL in promoting the activation of brown fat, EE, and weight loss was higher in mice housed at 30 °C compared with mice housed at 22 °C, suggesting that drug efficiency could be also affected by environmental temperature [ 76 ]. The efficacy of CL appears also to be reduced in the presence of obesity-associated low-grade inflammation [ 77 , 78 ], as obese mice with adipose tissue dysfunction and increased proinflammatory cytokines showed impaired β3-AR sensitivity to CL, leading to catecholamine resistance and reduced EE [ 91 ].
Desensitization of β3-AR occurs through a complex pathway involving exchange protein directly activated by cAMP EPAC and Ras-related protein 2 A RAP2A [ 78 ]. Under physiological conditions, β3-AR expression is regulated by CEBPα, which is a transcriptional factor also involved in the differentiation of new adipose cells as previously discussed [ 41 , 79 ].
Beyond promoting the resistance to adrenergic stimuli, EPAC is also known to foster resistance to leptin [ 80 ], an adipocyte-derived hormone recently identified as a novel thermolipokine and whose altered signalling may contribute to metabolic dysfunction [ 81 ].
Indeed, administration of the EPAC inhibitor ESI in obese mice restored β3-AR expression in WAT, increased EE, and promoted weight loss, thus suggesting that a functional β3-AR signalling is crucial for the maintenance of EE balance.
Notably, these benefits were also accompanied with an increased responsiveness to CL, together with the activation of hormone-sensitive lipase HSL in WAT, a known target of adrenergic signalling [ 78 ]. Mirabegron was the first highly selective β3-AR agonist approved as a therapy against overactive bladder [ 82 ].
Beyond this therapeutic indication, recent findings have highlighted a metabolic benefit of this drug, even though few studies have investigated its effects on weight control. observed that when administered daily in young healthy women, mirabegron acutely increased the metabolic activity of BAT, as revealed by the raise of [18F]fluoro-ddeoxy-d-glucose uptake, together with the amelioration of EE [ 11 ].
The metabolic benefits of mirabegron also extended after 4 weeks of therapy, showing an increase of the amount of BAT in association with the amelioration of lipid profile, insulin sensitivity, and insulin secretion [ 11 ].
Noteworthy, these outcomes were more evident in subjects with low amount of BAT at baseline, even though the beneficial responses were not accompanied by changes in body weight and composition [ 11 ].
Similarly, Finlin et al. reduced HbA1c levels, increased insulin sensitivity, improved β-cell function without a significant body weight loss [ 83 ].
The same authors also illustrated that these metabolic effects were achieved by the restoration of healthy adipose tissue together with enhanced activation of the beigeing process in subcutaneous WAT depots, an event probably driven by resident macrophages [ 83 ]. Considering that the amount of BAT has been generally found to be inversely correlated with BMI [ 6 ], stimulation of BAT activation and differentiation with mirabegron may have promising effects in the amelioration of energy balance of obese patients.
However, the absence of significant weight-lowering effects observed in obese patients makes this compound less attractive at present. Furthermore, longer-term studies are needed, especially in patients with higher BMI at baseline.
Further studies should also elucidate the role of potential catecholamine resistance in the long term in the therapeutic response to mirabegron [ 78 ].
During the past few decades, growing interest has been directed towards a possible therapeutic use of thyroid hormones THs and their mimetics in several dysmetabolic conditions i.
dyslipidaemia, liver diseases and weight management as well, leading to the development of new promising compounds [ 84 , 85 ]. The effects of THs on metabolic homeostasis were long observed clinically in patients suffering from hyperthyroidism, showing important reductions in body weight, cholesterol levels and, in certain cases, BAT activation [ 86 ].
However, the excess of THs is associated with symptoms and signs resulting from increased adrenergic stimulation which may lead to complications, such as tachycardia, atrial fibrillation, osteoporosis, muscle weakness, and anxiety, in the long term [ 87 ]. THs were formerly thought to promote their effects on energy homeostasis by directly acting on peripheral tissues, such as BAT and WAT, muscle, heart, and liver [ 88 ].
However, several data indicate that THs modulate thermoregulatory control during shivering and non-shivering cold adaptation and food intake, EE, and body weight by acting, to a large extent, at the central level [ 88 , 89 ].
Indeed, previous findings revealed that central administration of triiodothyronine T3 in rats resulted in increased EE by stimulating BAT thermogenesis and WAT browning via AMPK-dependent mechanism and upregulation of UCP1 [ 89 ] Figs. The thermogenic actions of central administration of T3 on BAT and WAT are brought about by suppressing AMPK activity in the ventromedial nucleus of the hypothalamus VMH.
Indeed, genetic ablation of AMPK in the VMH mimics the effects of T3 in the VMH by enhancing BAT thermogenesis and WAT browning [ 89 ]. Moreover, the critical role of UCP1 in mediating T3-induced increase in EE has been shown by several studies in which pharmacological or genetic deletion of UCP1 completely blunted the thermogenic action of central T3 [ 89 ].
THs activate the specific intracellular receptors TRs able to act as transcriptional factors by interacting with coactivators, corepressors, and thyroid response elements on DNA sequences.
Two isoforms of TRs have been identified: TRα, mainly located in heart, bone, and brain, and TRβ, principally expressed in tissues with endocrine and metabolic control such as liver and pituitary gland.
However, recent findings showed that TRβ is the major isoform mediating T3 actions on multiple metabolic pathways in WAT, including glucose uptake and consumption, de novo lipogenesis, and both UCP1-dependent and -independent thermogenesis [ 92 ].
However, these compounds and other novel analogues i. GC have shown to ameliorate EE and to trigger thermogenic responses when investigated both in vitro and in vivo. The first evidence of the thermogenic property of THs derive from in vitro studies where human multipotent adipose-derived stem cells treated with T3 for 3 days engaged browning differentiation as shown by the increase of UCP1 mRNA expression levels, mitochondrial biogenesis, and oxygen consumption rate [ 94 ].
Subsequently, Yau et al. demonstrated that T3-induced thermogenesis occurs via the stimulation of mitochondrial autophagy, activity, and turnover in both a BAT cell line and primary murine brown adipocytes [ 95 ].
In view of the thermogenic property of TH, different thyromimetics have been designed with the aim of developing new strategies to induce a BAT-like signature in vitro and for their possible use for the treatment of obesity and its associated metabolic disorders including dyslipidaemia and non-alcoholic fatty liver disease.
Ribeiro et al. observed that brown adipocytes isolated from hypothyroid mice treated with the TRβ1-selective thyromimetic, GC-1, showed an increased expression of UCP1, but less activation of adrenergic pathways when compared to brown adipocytes isolated from T3-treated mice, indicating that the stimulation of UCP1 and augmentation of adrenergic responsiveness are mediated by different TR isoforms in the same cells [ 96 ].
These effects were better elucidated by Lin and colleagues demonstrating that GC-1 robustly induced UCP1 mRNA and protein levels in mouse white adipocytes in a PKA-independent manner when compared to norepinephrine, thus indicating that GCrelated WAT browning occurs regardless of β-AR activation [ 97 ].
The thermogenic power of GC-1 was next confirmed in both bone marrow-derived macrophages and mouse preadipocytes where a significant upregulation of BAT markers i. proton-coupled amino acid transporter 2, PGC1α, PRDM16 after GC-1 administration was observed in an equipotent manner as with T3 stimulation [ 98 ].
Similar results have been found in adipocytes from murine BAT that showed increased mRNA expression of key thermogenic mediators i.
Pgc1β, Pparα, Pparδ, Cpt1, Ucp1, etc. after chronic treatment with GC, a TRβ-selective agonist [ 99 ]. The metabolic benefits of TRs agonists were established in animal models of hypothyroidism and hypercholesterolaemia in which the administration of GC-1 resulted in the amelioration of lipid profile and induction of UCP1 mRNA expression in BAT, while only minimally mediating synergism between TH and the sympathetic nervous system [ 96 ].
Similarly, experiments performed in rodents and primates revealed that both GC-1 and KB, another TH analog, favoured the improvement of EE in association with a decrease in fat mass and cholesterol plasma levels [ , ]. Considering the implication of these molecules in lipid metabolism, more recent preclinical studies examined the anti-obesity and anti-diabetic efficacy of the selective agonist GC GC-1 elicited a remarkable morphological change of subcutaneous WAT in terms of activation of the BAT-like programme of adaptive thermogenesis i.
increase of multilocular lipid droplet, UCP1, and mitochondrial DNA content together with fat loss, metabolic improvement, and recovery of cold tolerance when administered in genetically obese mice [ 97 ].
Nevertheless, the browning property of GC-1 appeared to be independent of BAT activity, since this molecule maintained the thermogenic ability in the setting of UCP1 deficiency [ 97 ].
The browning of WAT mediated by GC-1 has not been observed by other TR agonists, as reported after KB administration that caused a slight increase in metabolic rate in obese mice without changes in body temperature [ 97 ].
Notably, the effects of GC-1 appear to change according to the concentrations used. In fact, obese mice treated with low doses of GC-1 maintained impaired glycaemic control, while higher dose ameliorated glucose tolerance and insulin sensitivity in association with increased body temperature, thus suggesting that the induction of thermogenesis may play a role in mediating these beneficial metabolic effects [ 97 ].
The same molecule has also produced favourable results in rat models of type 2 diabetes T2D and obesity when administered by a nanochannel membrane device, providing a dramatic body weight reduction, normalization of cholesterol and glucose levels together with sustained expression of UCP1 in WAT [ ].
Thereafter, a second generation of TRβ-selective molecules with fold higher affinity compared to TRα has been characterized, GC, whose administration for 45 days 8.
PGC1α, UCP1 [ ]. One year later, Castillo et al. Taken together, these data give support to the awareness that TRβ agonists could eventually become good candidates in the therapeutic arsenal of obesity treatment by mediating the activation of several metabolic responses including thermogenic and browning processes.
Although both in vivo and in vitro data elucidated the role of THs and their mimetics in regulating BAT activity and browning, few human studies have investigated the effects of TH replacement therapy in this setting.
Of note, the thermogenic property of THs was well described in human observational studies regarding hypothyroidism, an endocrine disorder associated with increased cold sensitivity and reduced weight loss following calorie restriction.
In this condition, the recovery of euthyroidism through TH replacement therapy i. levothyroxine was noted to significantly increase cold-induced thermogenesis together with enhanced EE [ , ]. Similarly, patients with thyroid carcinoma under levothyroxine-based therapy in the post-surgery period showed an enhanced glucose uptake in the BAT during cold exposure [ ].
Nevertheless, severe cold intolerance may occur in some hypothyroid patients on levothyroxine therapy with residual symptoms [ ]. For this reason, a recent study investigated whether this limitation may be overcome by liothyronine.
Indeed, hypothyroid female patients treated with liothyronine showed a reduction of the drop in skin temperature during cold stimulation in both supraclavicular and sternal areas and increased body temperature, thus suggesting that despite restoring the euthyroid condition, levothyroxine therapy exhibited abnormal dermal heat loss and attenuated BAT activation as a sign of deficient T3 receptor action [ ].
Farnesoid X receptor FXR is a bile acid-activated nuclear receptor, mainly expressed in the liver and intestine playing a major role in regulating bile acids and lipid metabolism.
Different clinical trials have reported the effectiveness of FXR modulators in chronic liver diseases and dysmetabolic conditions i. obesity, metabolic syndrome, lipodystrophy, etc. Recent findings have also described that FXR may exert actions in extrahepatic tissues such as the kidney, adrenal glands, vascular walls, and adipose tissues.
The metabolic role of FXR was better clarified in mice in which whole-body genetic abrogation of this receptor induced glucose and insulin intolerance, together with impaired adipogenesis in WAT and severe cold intolerance [ , , ].
Conversely, when FXR knockout mice were exposed to energy overload, a protection from diet-induced obesity and attenuated adipose tissue expansion were observed [ ].
In addition to these contrasting results, the direct activity of FXR on BAT function remains ambiguous, even though Yang et al. have recently demonstrated that the expression of this receptor markedly decreased upon cold exposure in murine BAT [ ]. The same authors also highlighted that the adipose tissue-specific overexpression of FXR induced a pronounced whitening of BAT and downregulation of mitochondrial functions, thus suggesting that FXR could modulate thermogenesis in a tissue-specific manner [ ].
Considering the emerging role of FXR in controlling whole-body energy homeostasis, adipogenesis, and BAT function, many attempts have been made to develop new pharmacological compounds targeting FXR. Among the plethora of FXR ligands developed, INT, a semisynthetic bile acid derivative able to activate both FXR and takeda G protein-coupled receptor 5 TGR5 , has been shown to activate different metabolic pathways in vitro.
Corroborating results were also obtained by farnesol, a natural carbon organic compound found in many essential oils, whose activity increased UCP1 protein levels in both 3T3-L1 adipocytes and human mesenchymal stem cells [ ], and ultimately was found to induce thermogenesis via activation of AMPK [ ].
Fexaramine was the first gut-restricted FXR agonist with numerous metabolic benefits including WAT browning. In fact, fexaramine-based therapy limited diet-induced weight gain together with enhanced EE and body temperature when administered in obese mice [ ].
Furthermore, this molecule has been shown to increase energy utilization by BAT and to upregulate the expression of several BAT activators i. PGC1α, PGC-1β , as well as of their target genes involved in thermogenesis, mitochondrial biogenesis and fatty acid oxidation [ ].
The same therapy reduced visceral fat depots, enhanced lipolytic rates and promoted WAT browning in obese mice as compared to untreated controls [ ]. Conversely, the chronic administration of a synthetic FXR agonist GW accentuated body weight gain and glucose intolerance in high-fat diet HFD -induced obese mice in association with a decreased bile acid biosynthesis and EE.
In addition, worsening of the changes in liver and adipose tissue was observed after GW administration [ ]. GW appears also to control energy homeostasis by stimulating thermogenesis through the activation of FXR in brain areas as observed in a mouse model.
After intracerebroventricular infusion of GW, mice showed important histological remodelling of BAT i. increase of lipid droplet size , which occurred via the control of hypothalamic sympathetic tone [ ].
However, these responses were absent in mice with FXR deficiency indicating target selectivity of the compound [ ]. The modulation of FXR activity was also investigated by using natural thermogenic nutrients such as bile acids. Diet supplementation with chenodeoxycholic acid CDCA or the bile acid-binding resin colestimide, which favours bile acids synthesis, in mice showed an anti-obesity efficacy associated with the increase of UCP1 and PGC1α mRNA expression levels in BAT depots [ ], reduced food intake and increased lipid oxidation in WAT [ ].
Similarly, farnesol, an isoprenoid present in essential oils, has been shown to ameliorate obesity and diabetes [ ], and ultimately was found to burn fuel energy by triggering thermogenic responses in HFD-induced obese mice [ ].
Notably, farnesol also induced thermogenic factors via AMPKα phosphorylation in BAT under cold acclimation Fig. In this setting, mice also showed weight loss and reduced BAT and WAT depots as compared to untreated mice [ ].
Further, in vivo studies have also observed that obeticholic acid OCA , a bile acid analogue originally developed for treating liver fatty diseases, exerts a browning effect on WAT.
When administered in hyperphagic mice, OCA ameliorated body weight and hepatic steatosis by activating endogenous BAT, but without altering fatty acid oxidation [ ].
Based on thermogenic control of bile acids, recent studies have investigated the effects of bile acid mimetics in the regulation of BAT function. Indeed, HFD rabbits treated with INT showed a reduction of visceral adipose tissue accumulation, hypercholesterolaemia and non-alcoholic steatohepatitis combined with the upregulation of mitochondrial and BAT-specific markers [ ].
Nowadays, few studies have investigated the potential role of FXR—bile acids axis in regulating EE and browning in humans.
For instance, modifications of circulating bile acids have been shown to correlate with changes in energy and substrate metabolism upon bariatric surgery [ , ]. Other candidates able to target FXR have been examined in clinical trials i.
OCA, WAY , showing positive results in the management of cholestatic liver diseases and metabolic syndrome [ ], but the effects on thermogenesis and browning processes are still unexplored.
Glucagon-like peptide 1 GLP-1 is an incretin produced by enteroendocrine L-cells in response to nutrient ingestion that has multiple pleiotropic effects, including the stimulation of insulin release from pancreatic β-cells, inhibition of glucagon secretion [ 51 , 52 , ], regulation of food intake by promoting satiety and fullness [ ], delay of gastric emptying [ ], and control of energy balance [ ].
GLP-1 release from the ileum depends on the composition and size of meals and can be impaired in both obesity and T2D [ , ]. The insulin-secretagogue effects of GLP-1 are mediated by GLP-1 receptors GLP-1R , which belong to the class B family of 7-transmembrane-spanning, heterotrimeric G protein-coupled receptors expressed in several tissues, including the pancreatic islet, kidney, heart, stomach, intestine, muscle, and central and peripheral nervous systems [ , , , , ].
However, data from a recent single-cell RNA sequencing analysis investigating the whole-body distribution of GLP-1R have revealed no expression of such receptors in both human and mouse WAT [ , ].
GLP-1 has a short half-life due to its rapid degradation induced by dipeptidyl peptidase 4 DPP4 , leading to the generation of largely inactive forms GLP—36 amide or GLP—37 [ , ]. Therefore, several structurally optimized GLP-1 analogues with improved bioavailability and sustained action have been developed for clinical use.
Among these, liraglutide and exendin-4 have been investigated specifically for their effect on EE. Although exenatide and liraglutide differ in terms of amino acid sequence homology with native GLP-1, both compounds showed similar thermogenic capabilities in vitro. Similar results have also been reported for exendin-4, which increased the expression of PGC1α and UCP1 via activation of SIRT1 when added to 3T3-L1 adipocytes cultures [ ].
These results highlight that SIRT1 is a key bioenergetic regulator shared by both β3-AR- and GLP-1R-mediated thermogenic signalling pathways. The peripheral and central effects of GLP-1 were elucidated in vivo by using GLP-1R-deficient models and long-acting GLP-1RA.
Firstly, Heppner et al. demonstrated that GLP-1R-knockout mice fed an HFD exhibited a blunted response to liraglutide in terms of reduced EE and weight loss when exposed to both noradrenaline and cold temperature [ ]. These results indicate the potential involvement of endogenous GLP-1R signalling in the maintenance of both adaptive thermogenesis and body weight.
EE modification appears to require the activation of GLP-1R signalling in certain brain areas [ ]. The GLP-1R is highly expressed in the hypothalamus, particularly in anorexigenic pro-opiomelanocortin POMC neurons within the arcuate nucleus ARN and in other areas closely associated with BAT activity i.
dorsomedial hypothalamus DMH and medial preoptic area MPOA [ ]. Indeed, rats with GLP-1R knockdown within DMH develop an obesogenic phenotype due to reduced EE and BAT thermogenesis, with a concomitant increase in hepatic steatosis, insulin resistance, and body weight [ ].
Central infusion of exendin-4 in mice induced an increase of sympathetic activity targeting BAT, with increased UCP1 protein expression, and improved TG clearance by robustly increasing TG uptake by brown adipocytes, while this disappeared in the presence of obesity [ ].
The amelioration of lipid turnover under pharmacological stimulation of central GLP-1R occurred through a shift in energy metabolism from carbohydrates to fatty acids as the prevalent energy source [ ], which confirmed previous data showing that chronic exposure to GLP-1 increased the rate of fatty acid oxidation by BAT [ ].
Interestingly, in vivo investigations have described a new hypothalamic pathway that, when activated by liraglutide, was found to trigger BAT thermogenesis, and browning of WAT in an AMPK-dependent manner, regardless of nutrient intake [ 30 ].
A single intracerebroventricular administration of liraglutide reduced food intake and body weight and increased BAT thermogenesis and UCP1 protein expression in WAT [ 30 , ]. Similarly, mice challenged with a high-fat and high-sugar diet that were exposed peripherally to liraglutide, beyond changes in eating behaviour, displayed a significant increase in BAT activation and brown fat markers in skeletal muscle and activation of adaptive thermogenesis in WAT via the AMPK—SIRT1—PGC1α pathway Fig.
Consistent with these findings, selective in vivo ablation of GLP-1R in the neuronal circuits of both DMH and VMH nuclei blunted the effect of liraglutide on body weight, in addition to reducing the thermogenic and browning action mediated by liraglutide and exendin-4 [ 30 , , ]; these observations suggest that energy dissipation might contribute to weight loss in response to these agents.
Despite an increasing body of evidence on the ability of incretin mimetics to elicit BAT responses through both direct and indirect mechanisms, to date few studies have explored the molecular machinery of adipocyte browning induced by GLP-1RA.
Recently, liraglutide was shown to participate in mitochondrial fat oxidation by modulating two key enzymes downstream of AMPK signalling i. long-chain acyl-CoA dehydrogenase [LCAD] and acetyl-CoA carboxylase-2 [ACC2] , reducing fat accumulation in association with suppression of adipogenesis, particularly in visceral fat regions of HFD diabetic mice [ ].
These events were achieved through induction of UCP1 and PRDM16, key mediators of browning, together with activation of the deacetylase SIRT1 [ ], whose involvement in browning was noted [ , , , ].
Furthermore, when GLP-1R were chronically activated by liraglutide in mice, an increase in the number of mitochondria was observed in both subcutaneous and visceral depots in association with the formation of new beige adipocytes through the activation of soluble guanylyl cyclase sGC and protein kinase G 1 PKG1 [ ], which appeared to be novel mediators of the browning process in WAT depots [ , ].
The extent of signalling activation by both receptors appeared to change in a tissue-dependent manner: rats exhibited a higher phosphorylation rate of PKA in the liver together with enhanced expression of PPARα and PPARγ, and improved lipid trafficking and resting EE after combined therapy [ ]; in contrast, PKA was activated to a lesser extent in BAT and WAT in association with an increase of thermogenic factors e.
cytochrome c oxidase subunit 4 isoform 1 and decrease of lipogenic mediators i. PPARα, PPARγ Fig. In this model, the combined therapy shifted the energy balance towards oxidative processes, enhancing peripheral pathways promoting fat mass reduction, liver fat content reduction, and an overall improved metabolic profile [ ].
On the basis of these preclinical findings, a combined therapy with GLP-1RA and β3-AR agonists could have potential on metabolic outcomes, even though its efficacy in human obesity is warranted. More recently, new GLP-1RAs, such as semaglutide or supaglutide, have been developed showing similar or even greater benefits in terms of weight loss, induction of EE and browning of WAT in mice models of obesity, thus further corroborating previous evidence regarding the thermogenic role of GLP-1R activation [ , ].
Taken together, these results suggest that, at least in experimental animal models, the body weight-lowering effect of GLP-1RA, particularly of liraglutide, may occur by increasing EE through a thermogenic mechanism, independent of changes in caloric intake.
However, clinical data have not always supported these promising experimental findings, as GLP-1RA-based therapy was found to lower energy intake without affecting EE or adaptive thermogenesis in humans [ 44 ]. Although it was found that body weight reduction induced by GLP-1RA may occur at least partially through increased thermogenesis and EE in experimental animals, conflicting results have been obtained so far in humans.
It was reported that long-term exposure to exenatide or liraglutide in individuals with obesity and T2D resulted in a reduction in BMI and fat mass and an increase in EE [ 30 ]. Nevertheless, these findings are in contrast with previous results showing that the anti-obesity efficacy of short-term therapy with GLP-1RA was not accompanied by an improvement in EE [ , , ].
Indeed, evidence of the thermogenic effect of GLP-1RAs in humans was provided by performing a tomography scanning of healthy volunteers treated with exendin-4 for 12 weeks, showing an increase in BAT glucose uptake [ ].
Although effects on EE could not be detected after 12 weeks, they were reported in another study in T2D patients when exendinbased therapy was extended at one year [ 30 ].
Similar effects were also observed after administration of liraglutide [ 30 , ], a drug currently used for both T2D and obesity management [ , ]. Although liraglutide shows an improved bioavailability as compared to native GLP-1, it requires daily injections, thus potentially reducing therapy adherence [ ].
The longer acting analogue semaglutide, with once-weekly administration and greater weight-loss efficacy as compared to liraglutide [ ], may provide greater clinical benefit. Semaglutide shows similar thermogenic capabilities in experimental animals [ ] and appears to be the most effective in reducing weight among the various GLP-1RA, with a mean weight loss of However, the potential thermogenic effects of semaglutide have not been yet elucidated in humans.
A clinical trial to clarify the role of BAT activation in mediating the weight loss induced by semaglutide in obese patients is being carried out, with results expected to be available in [ ]. Glucose-dependent insulinotropic polypeptide GIP is another insulinotropic protein secreted by enteroendocrine K cells of the small intestine acute ingestion of dietary nutrients [ ].
Evidence from in vivo studies reported conflicting results regarding GIP release in T2D, with some studies showing enhanced [ ] or unchanged secretion [ , ]. The release of GIP is also modified under cold acclimatation, as observed in rats in which chronic cold exposure significantly augmented circulating levels of GIP in association with an increase in BAT mass [ ].
GIP acts through the activation of G protein-coupled receptor GIPR , whose expression has also been observed in extra-pancreatic tissues, including the hypothalamus and adipose tissue, suggesting that it controls systemic metabolism beyond insulin secretion [ ].
The distribution of GIPR at the adipose tissue level was already established, particularly in preadipocytes [ ], in which GIPR expression levels are enhanced after adipocyte commitment [ ]. However, the expression of GIPR in both mouse and human WAT remains questionable, since analysis of single-nucleus RNA sequencing has recently found GIPR to be predominantly localized in nonadipocyte cells with prevalent expression in pericytes and mesothelial cells [ , ], suggesting that the effects of GIP on adipocytes could be exerted through indirect mechanisms.
Notably, GIPR expression levels in adipose tissue were found to be negatively correlated with adiposity and positively associated with insulin sensitivity [ ].
The role of GIPR signalling in the regulation of both systemic and adipose tissue metabolism is not fully elucidated, since transgenic animal models produced conflicting results. Mantelmacher et al. The latter data suggest that increased infiltration of immune cells in adipose tissue could affect the adaptive thermogenesis of BAT through a GIP-dependent mechanism.
More recently, new findings suggest that the stimulation of GIPR could promote BAT function and thermogenesis, as well as the beigeing process, in human WAT precursors, in association with the amelioration of lipid metabolism [ ].
These responses appear to require PKA activation [ ], which is also involved in β3-adrenergic-mediated thermogenesis, as previously discussed [ 65 ]. Considering the favourable metabolic effects of both GLP-1 and GIP, combined therapies with both these incretins have been investigated to improve the efficacy of obesity treatments.
Multi-receptor pharmacology is at the forefront of next-generation therapies for the treatment of metabolic diseases. Indeed, the synergic effect of GLP-1 and GIP has already been investigated in vivo with the intra-cerebroventricular co-administration of both incretin hormones that significantly decreased body weight by reducing food intake through the central activation of anorexigenic POMC neurons located in the ARN of the hypothalamus [ ].
Nevertheless, this weight-lowering effect was lost when either peptide was infused alone, suggesting that simultaneous central activation of GLP-1R and GIPR is essential for regulating energy balance [ ].
It is already known that the ARN plays a critical role in metabolic regulation through a direct excitatory glutamatergic projection to the paraventricular nucleus PVN of the hypothalamus [ ], which in turn modulates BAT activation and thermogenesis through sympathetic activity [ ]. Among these, NNC and tirzepatide showed improved efficacy in terms of EE, insulin sensitivity and weight loss, as compared to single GLP-1R agonism in a model of diet-induced obesity [ , ].
Currently, it is not fully clear whether these beneficial effects are promoted by GLP-1R, GIPR or by simultaneous and possibly unbalanced activation of these receptors. Despite losing equivalent body weight, obese mice treated with tirzepatide showed a greater amelioration of systemic insulin sensitivity when compared to obese mice treated with GLP-1R monoagonist semaglutide , an effect mediated by enhanced glucose disposal in both WAT and BAT [ ].
The weight-independent insulin sensitization induced by tirzepatide appeared to be due to the engagement of GIPR. Indeed, similarly to long-acting GIPR agonist LAGIPRA , tirzepatide favoured similar protection from obesity and insulin resistance, together with increased catabolism of glucose, lipids, and branched-chain amino acids BCAA in BAT.
glutamate, alanine, etc. principally involved in cold-induced thermogenesis [ ]. Altogether, these data define, for the first time, the critical role of GIPR activation in mediating the metabolic outcomes of tirzepatide regardless of its weight-lowering action, highlighting an effect that involves the activation of a thermogenic-like amino acid signature in BAT.
The clinical relevance of both NNC and tirzepatide was also investigated in clinical studies. While no significant improvement between NNC and liraglutide in terms of reduction of hyperglycaemia and weight loss was observed in patients with T2D [ ], tirzepatide showed greater benefits on both these endpoints compared to semaglutide [ ], thus becoming the most effective anti-obesity drug currently available [ ].
Differences between these dual agonists could be explained by considering their affinity for the GLP-1R and GIPR. NNC equally binds both GLP-1R and GIPR, whereas tirzepatide acts as an unbalanced agonist showing higher potency for GIPR rather than for GLP-1R [ , ].
The partial agonism of tirzepatide could be linked to its ability, when compared to native GLP-1, to induce less β-arrestin recruitment, a key signalling protein mediating GLP-1R internalization, thus resulting in higher cell-surface GLP-1R levels and enhanced beta-cellular responses in terms of insulin secretion [ ].
Although EE was not evaluated in the latest clinical trials, a study measuring food intake and calorie consumption in overweight patients using tirzepatide is currently ongoing [ ]. In accordance with data obtained by studies in rodents [ , ], Pirro et al.
observed that tirzepatide reduced circulating BCAA in patients with T2D, and this effect was strongly associated with biomarkers indicative of enhanced insulin sensitivity [ ].
Glucagon is a well-known peptide secreted by pancreatic α-cells that can correct hypoglycaemia by increasing hepatic glucose production [ 54 ]. Glucagon also exhibits pleiotropic properties, such as regulation of feeding behaviour i.
satiety and fullness [ ], lipid homeostasis [ ], insulin secretion, and EE [ ]. Glucagon acts mainly through a G-coupled receptor GCGR whose expression was found in hepatocytes, where this hormone displays principal functions in terms of stimulation of both gluconeogenesis and glycogenolysis [ ].
GCGR was also identified in peripheral tissues i. kidney, heart, spleen, thymus, stomach, duodenum, brain [ ] and its transcript has recently been reported in adipose tissue, particularly in mature adipocytes from both WAT and BAT samples [ ].
GCGR distribution and activity in adipose tissue was established several years ago demonstrating the presence of high-affinity glucagon-binding sites in the soluble membranes of human fat cells [ ]; in this setting, glucagon exhibited direct lipolytic action in a concentration range of 10 —6 —10 —8 M [ ] through a cAMP-dependent mechanism [ ].
An effect of glucagon on thermogenesis was described several decades ago [ , ], showing a decrease in body weight with an additional increase of EE following subcutaneous injection of glucagon in obese rats [ ].
These effects seem to involve a BAT-dependent mechanism, since increases in temperature [ ], blood flow, and cold-induced BAT mass [ 55 ] were observed following glucagon-based therapy.
However, consistent data have indicated that glucagon could also affect heat production and BAT metabolism through indirect mechanisms. Of note, the role of glucagon in the regulation of thermogenesis has been better clarified in mice with genetic deletion of GCGR, in which a blunted cold-induced activation of adaptive thermogenesis and browning together with the reduction of PKA substrates phosphorylation in WAT was observed [ 31 ].
Based on these considerations, glucagon could be a potential molecule to counteract obesity through the activation of EE and BAT, even though the well-known hyperglycaemic excursions associated with this hormone [ ] make it potentially harmful if used as a monotherapy for obese subjects with impaired glucose regulation.
Therefore, combined therapies with hypoglycaemic agents, such as incretin mimetics, have been further explored. This peptide harbours modifications that allow it to maximize stability and efficacy in terms of resistance to cleavage by DPP4 and increased half-life, with a retained greater affinity for GCGR [ ].
In the same study, O 2 consumption and CO 2 production were measured through indirect calorimetry and revealed an increased EE in mice treated by the dual agonist, suggesting that a metabolic shift towards heat production could contribute to the pronounced weight-lowering effect [ ]. The mice did not exhibit changes in physical activity or food intake when on dual agonist therapy compared with untreated littermates, suggesting that the increased EE occurred mainly through increased basal metabolism and thermogenesis [ ].
Interestingly, the dual agonist-induced fat loss was observed in visceral depots in which adipocytes acquired a small phenotype in association with increased phosphorylation of HSL suggesting that enhanced lipolysis could have a role in fat reduction [ ].
The key component that primarily triggers fat burn is the glucagon-related sequence within the dual agonist peptide, since this hormone is known to enhance lipolysis, as reported by previous studies in which glucagon administration in human adipocytes induced a dose-dependent increase in lipolysis rates [ , , ].
The synergistic contributions of combined GLP-1R and GCGR activation were also evaluated in GLP-1R-null mice maintained on an HFD, in which the dual agonist led to reduced body weight and adiposity due to an anorexic effect while hyperglycaemia was retained; this highlights that a GLP-1 fraction inside the chimeric compound is needed to protect against glucagon-induced hyperglycaemia [ ].
Similar findings were observed after treatment with cotadutide, another dual agonist of both GLP-1R and GCGR, whose administration increased EE in DIO mice, with greater weight loss and a similar glucose-lowering effect compared with liraglutide [ ].
The weight-lowering effects of cotadutide was also replicated in non-human primates, indicating that this drug maintains similar benefits in different species [ ].
Furthermore, cotadutide produced body weight loss, amelioration of glucose tolerance, reduced liver fat content, and increased mRNA expression of the brown thermogenic genes Ucp1 , Pgc1α , and β3-AR in an obese mouse model with leptin deficiency [ ].
These beneficial responses were not sustained with single-agonist administration, suggesting that a specific mechanism involved in BAT activation was induced only when both GLP-1R and GCGR signalling were chronically activated [ ].
Considering these results in preclinical models, the simultaneous activation of GLP-1R and GCGR could be a promising strategy to improve both adiposity and lipid profile without a worsening of glucose levels.
In this regard, when GLP-1R signalling was abrogated, hyperglycaemic excursions were observed, indicating that GLP-1R activation is essential in offsetting glucagon-induced hyperglycaemia. The synergic effects of GLP-1 and glucagon were also explored in studies in human healthy volunteers in which combined infusion of low doses of both hormones increased EE to a greater extent than what was achieved with either peptide infused alone [ ].
The first of this new class of anti-diabetic agents tested in humans was oxyntomodulin, a amino acid hormone secreted by the fundic cells of the colon [ ]. When administered to obese subjects, this peptide successfully reduced calorie intake and increased EE, resulting in negative energy balance and significant weight loss [ ].
These promising results encouraged the development of more suitable synthetic dual agonists, such as cotadutide and the more recent mazdutide, which successfully reduced body weight in exploratory analysis of obese and T2D patients [ , ]. The metabolic benefits of these triple agonists were found to be higher than mono and dual therapy [ , ].
Curiously, cold-evoked BAT thermogenesis was unaffected by lesions of the PVH Lu et al. In contrast, activation of neurons in the PVH has recently been demonstrated to inhibit BAT SNA and BAT thermogenesis Madden and Morrison, Although activation of PVH neurons could attenuate the increases in BAT SNA and BAT thermogenesis evoked by injections of NMDA into the rRPa, those resulting from bicuculline injections into rRPa were unaffected by disinhibition of PVH neurons Figure 6 B , consistent with the PVH-evoked inhibition of BAT SNA being mediated by GABA A receptors in the rRPa.
That neurons in the PVH provide an inhibitory influence on BAT SNA is also supported by the observations that NPY presynaptically inhibits GABA release onto PVH neurons Cowley et al. These apparent controversies in the relation of PVH neurons to BAT thermogenesis, particularly during fever, might be explained by the presence of subpopulations of PVH neurons mediating contrasting effects or by a role of PVH neurons during fever that involves the stimulation of other fever-supporting effector systems such as the cutaneous vasculature or hormone release, whereas the inhibition of BAT thermogenesis by PVH neurons contributes to a non-febrile homeostatic function.
Figure 6. Disinhibition of neurons in the paraventricular hypothalamic PVH nucleus inhibits the increases in BAT sympathetic nerve activity SNA evoked by cooling, but not those evoked by disinhibition of neurons in the rostral raphe pallidus rRPa.
A Nanoinjection of bicuculline BIC into the PVH completely reversed the increases in BAT SNA, BAT temperature TBAT , expired CO 2 Exp CO 2 produced by whole body cooling. Core body temperature TCORE was also reduced; heart rate HR and arterial pressure AP were increased by BIC in PVH.
B The increases in BAT SNA, TBAT, expired CO 2 , TCORE, HR, and AP evoked by nanoinjection of BIC into the rRPa are not affected by nanoinjection of BIC into the PVH.
Controversy also exists concerning the role of melanocortin receptor activation in the PVH in energy expenditure and activation of BAT thermogenesis. Selective rescue of melanocortin-4 receptor MC4R expression in neurons of the PVH and the medial amygdala in mice lacking expression of MC4R failed to normalize elevate their oxygen consumption to wild-type levels Balthasar et al.
Based on these data it was suggested that PVH MC4Rs do not mediate the energy expenditure effects of melanocortins. In contrast, other groups have demonstrated that microinjection of melanocortin receptor agonists into the PVH increases core and BAT temperatures Song et al. These effects of melanocortin receptor activation could be mediated by activation of presynaptic MC4Rs, which have been shown to potentiate GABAergic inputs to PVH neurons Cowley et al.
Indeed, this explanation would reconcile this controversy, since the rescue of MC4R in the study of Balthasar et al. would only rescue the postsynaptic MC4R in PVH neurons and not those located presynaptically and potentially responsible for the effects of exogenously administered melanocortin receptor agonists.
This explanation is also consistent with our data indicating that the activity of neurons in the PVH is inhibitory to BAT SNA. The physiological conditions which would stimulate the BAT sympathoinhibitory output from the PVH are unknown, but may include hypoglycemia and chronic intermittent hypoxia.
Another interesting possibility is that neurons in the PVH provide a tonic inhibition of BAT thermogenesis and release from this inhibition under specific conditions, such as changes in dietary composition, may activate BAT SNA and BAT energy expenditure.
Inactivation of neurons in the vicinity of the pontine retrorubral field produced a similar stimulation of BAT thermogenesis Shibata et al. Neither the exact location of the neurons mediating this inhibition nor the physiological basis for its control has been determined.
The VLM and the NTS contain neurons that comprise fundamental cardiovascular and respiratory regulatory circuits, including the baroreceptor and arterial chemoreceptor reflex pathways regulating local vasoconstrictor and cardiac sympathetic premotor neurons, as well as the respiratory generating network and its regulation by central chemoreceptors.
A role for neurons in these same regions in regulating BAT thermogenesis has been recently described Cao et al. The VLM region from which inhibitions of BAT SNA could be evoked corresponds to the locations of the A1 and C1 cell groups in the region ventral to the nucleus ambiguous.
Similar inhibitions of BAT SNA were also produced by bilateral injections of bicuculline into the medial NTS Figure 7 B. However, application of leptin and TRH into the NTS elicits increases in BAT temperature Hermann et al. Whether the inhibitions of BAT thermogenesis evoked throughout this rostro-caudal extent of the VLM represent activation of a homogeneous inhibitory mechanism or stimulations of different mechanisms at different rosto-caudal levels remains to be determined, as do the mechanisms through which the VLM and the NTS inhibitions of BAT SNA is effected and the physiological circumstances under which such inhibitions of BAT thermogenesis would normally be elicited.
In this regard, both hypoxia and hypoglycemia are strong inhibitory signals for BAT thermogenesis see above and the commissural NTS contains second-order chemoreceptor sensory neurons mediating an inhibition of BAT SNA Madden and Morrison, , and the VLM contains neurons sensitive to glucopenia Ritter et al.
Figure 7. Activation of neurons in the ventrolateral medulla VLM or in the nucleus of the solitary tract NTS inhibits BAT sympathetic nerve activity SNA and BAT thermogenesis. A Cooling-evoked increases in BAT SNA, BAT temperature TBAT , and expired CO 2 Exp CO 2 were reversed following unilateral nanoinjection of either the glutamate receptor agonist, NMDA, or the GABAA receptor antagonist, bicuculline BIC , into the rostral VLM RVLM.
Core temperature TCORE was also reduced by activation of RVLM neurons. B Increases in BAT SNA, TBAT, and ExpCO 2 evoked by BIC nanoinjection into the rostral raphe pallidus rRPa were reversed by bilateral nanoinjections of BIC into the medial NTS.
Modified with permission from Cao et al. Brown adipose tissue thermogenesis is regulated primarily by a core thermoregulatory neural network which responds to the feedforward afferent signals from cutaneous and core body thermoreceptors and to feedback signals from brain thermosensitive neurons to alter the level of activation of the sympathetic outflow to BAT.
We have summarized the research leading to a model Figure 1 of the thermoregulatory reflex pathway through which environmental cold stimulates thermogenesis and includes the influence on this thermoregulatory network of the pyrogenic mediator, PGE 2 , to increase body temperature during fever.
The cold thermal afferent circuit from cutaneous thermal receptors, through second-order thermosensory neurons in the dorsal horn of the spinal cord ascends to activate neurons in the LPBel which drive GABAergic interneurons in the MnPO to inhibit warm-sensitive, inhibitory output neurons of the MPO.
Through the resulting disinhibition of thermogenesis-promoting neurons in the DMH, BAT sympathetic premotor neurons in the rostral ventromedial medulla, including the rRPa, are stimulated to increase the excitation to and responsiveness of the spinal circuits controlling BAT SPN discharge to drive BAT thermogenesis.
Hypoxia and hypoglycemia are strong metabolic regulators of BAT thermogenesis. The activity of neurons in several brain regions can influence the level of BAT thermogenesis and thus could modulate the thermoregulatory control of BAT, although their discharge does not appear to be required to mediate the skin cooling-evoked stimulation of BAT thermogenesis.
These include an orexinergic excitation of BAT thermogenesis from neurons in the PeF-LH and inhibitory regulation of BAT thermogenesis by neurons in the PVH, the pontine retrorubral field, the VLM and the NTS. The authors declare that the research was conducted in the absence of any commercial or financial relationships that could be construed as a potential conflict of interest.
Support of the research that contributed to this review came from National Institutes of Health grants R01NS Shaun F. Morrison , R01DK Shaun F. Morrison , F32DK Christopher J. Madden , R56DK Christopher J. Madden , and an American Heart Association Scientist Development Grant Christopher J.
Almeida, M. Neural substrate of cold-seeking behavior in endotoxin shock. PLoS ONE 1, e1. Pubmed Abstract Pubmed Full Text CrossRef Full Text. Amini-Sereshki, L. Brain stem tonic inhibition of thermoregulation in the rat.
Pubmed Abstract Pubmed Full Text. Andrew, D. Spinothalamic lamina I neurons selectively sensitive to histamine: a central neural pathway for itch.
Bacon, S. Preganglionic sympathetic neurones innervating the rat adrenal medulla: immunocytochemical evidence of synaptic input from nerve terminals containing substance P, GABA or 5-hydroxytryptamine. Balthasar, N. Divergence of melanocortin pathways in the control of food intake and energy expenditure.
Cell , — Bamshad, M. CNS origins of the sympathetic nervous system outflow to brown adipose tissue. Bartness, T.
Sympathetic and sensory innervation of brown adipose tissue. Bautista, D. The menthol receptor TRPM8 is the principal detector of environmental cold. Nature , — Berthoud, H. Orexin inputs to caudal raphe neurons involved in thermal, cardiovascular, and gastrointestinal regulation.
Cell Biol. Blatteis, C. Autonomic thermoregulation after separation of the preoptic area from the hypothalamus in rats. Pflugers Arch. Boulant, J. Counterpoint: Heat-induced membrane depolarization of hypothalamic neurons: an unlikely mechanism of central thermosensitivity.
CrossRef Full Text. Temperature receptors in the central nervous system. The effect of spinal and skin temperatures on the firing rate and thermosensitivity of preoptic neurones. Bratincsak, A. Activation of brain areas in rat following warm and cold ambient exposure.
Neuroscience , — Evidence that peripheral rather than intracranial thermal signals induce thermoregulation. Buchanan, T. Hypothermia is critical for survival during prolonged insulin-induced hypoglycemia in rats. Caldeira, J. Bilateral lesion of hypothalamic paraventricular nucleus abolishes fever induced by endotoxin and bradykinin in rats.
Cammack, C. Excitation of rat sympathetic preganglionic neurones by selective activation of the NK1 receptor. Cannon, B. Brown adipose tissue: function and physiological significance.
Cano, G. Anatomical substrates for the central control of sympathetic outflow to interscapular adipose tissue during cold exposure. Cao, W. Medullary pathways mediating specific sympathetic responses to activation of dorsomedial hypothalamus.
Inhibition of brown adipose tissue thermogenesis by neurons in the ventrolateral medulla and in the nucleus tractus solitarius.
Brown adipose tissue thermogenesis contributes to fentanyl-evoked hyperthermia. Glutamate receptors in the raphe pallidus mediate brown adipose tissue thermogenesis evoked by activation of dorsomedial hypothalamic neurons.
Neuropharmacology 51, — Caterina, M. Impaired nociception and pain sensation in mice lacking the capsaicin receptor. Science , — Cerri, M. Activation of lateral hypothalamic neurons stimulates brown adipose tissue thermogenesis.
Corticotropin releasing factor increases in brown adipose tissue thermogenesis and heart rate through dorsomedial hypothalamus and medullary raphe pallidus.
Chen, X. Efferent projection from the preoptic area for the control of non-shivering thermogenesis in rats. The caudal periaqueductal gray participates in the activation of brown adipose tissue in rats. Christensen, C. Reversal of hypermetabolic brown adipose tissue in F FDG PET imaging.
Clapham, J. Central control of thermogenesis. Neuropharmacology doi: Colburn, R. Attenuated cold sensitivity in TRPM8 null mice. Neuron 54, — Conte, D. Do serotonin immunoreactive terminals innervate GABAergic neurons in the central autonomic area of the mouse spinal cord.
FASEB J. Coote, J. The response of individual sympathetic preganglionic neurones to microelectrophoretically applied endogenous monoamines. Brain Res. Cowley, M. Integration of NPY, AGRP, and melanocortin signals in the hypothalamic paraventricular nucleus: evidence of a cellular basis for the adipostat.
Neuron 24, — Craig, A. How do you feel? Interoception: the sense of the physiological condition of the body. A thalamic nucleus specific for pain and temperature sensation.
Quantitative response characteristics of thermoreceptive and nociceptive lamina I spinothalamic neurons in the cat.
Cypess, A. Identification and importance of brown adipose tissue in adult humans. de Lecea, L. II, Frankel, W. The hypocretins: hypothalamus-specific peptides with neuroexcitatory activity. de Menezes, R.
Cardiovascular and thermal responses evoked from the periaqueductal grey require neuronal activity in the hypothalamus. Deuchars, S. GABAergic neurons in the central region of the spinal cord: a novel substrate for sympathetic inhibition. Stimulation within the rostral ventrolateral medulla can evoke monosynaptic GABAergic IPSPs in sympathetic preganglionic neurons in vitro.
Dhaka, A. TRPM8 is required for cold sensation in mice. Dib, B. Thermoregulatory behaviour induced by intrathecal injection of substance P in the rat. Dimicco, J. The dorsomedial hypothalamus: a new player in thermoregulation.
Egawa, M. Effects of 2-deoxy-D-glucose on sympathetic nerve activity to interscapular brown adipose tissue. Lateral hypothalamic injection of 2-deoxy-D-glucose suppresses sympathetic activity. Neuropeptide Y suppresses sympathetic activity to interscapular brown adipose tissue in rats.
Elmquist, J. Distribution of Fos-like immunoreactivity in the rat brain following intravenous lipopolysaccharide administration. Mechanisms of CNS response to systemic immune challenge: the febrile response. Trends Neurosci. Fan, W. Thermogenesis activated by central melanocortin signaling is dependent on neurons in the rostral raphe pallidus rRPa area.
Freinkel, N. The hypothermia of hypoglycemia. Studies with 2-deoxy-D-glucose in normal human subjects and mice. Gavva, N. The vanilloid receptor TRPV1 is tonically activated in vivo and involved in body temperature regulation.
Golozoubova, V. UCP1 is essential for adaptive adrenergic nonshivering thermogenesis. Guieu, J. Effects of heating and cooling of the spinal cord on preoptic unit activity.
Gupta, B. Cold-sensitive afferents from the abdomen. Hany, T. Brown adipose tissue: a factor to consider in symmetrical tracer uptake in the neck and upper chest region. Imaging 29, — Hara, J. Difference in obesity phenotype between orexin-knockout mice and orexin neuron-deficient mice with same genetic background and environmental conditions.
Hermann, D. Afferent projections to the rat nuclei raphe magnus, raphe pallidus and reticularis gigantocellularis pars alpha demonstrated by iontophoretic application of choleratoxin subunit b. Hermann, G. Leptin and thyrotropin-releasing hormone: cooperative action in the hindbrain to activate brown adipose thermogenesis.
Hetherington, A. Hypothalamic lesions and adiposity in the rat. Hodges, M. Defects in breathing and thermoregulation in mice with near-complete absence of central serotonin neurons.
Hori, T. Capsaicin and central control of thermoregulation. Horn, T. Reduced febrile responses to pyrogens after lesions of the hypothalamic paraventricular nucleus. Hylden, J. Spinal lamina I projection neurons in the rat: collateral innervation of parabrachial area and thalamus.
Neuroscience 28, 27— Imai-Matsumura, K. Involvement of ventromedial hypothalamus in brown adipose tissue thermogenesis induced by preoptic cooling in rats. Ishiwata, T. Changes of body temperature and thermoregulatory responses of freely moving rats during GABAergic pharmacological stimulation to the preoptic area and anterior hypothalamus in several ambient temperatures.
Jancso-Gabor, A. Irreversible impairment of thermoregulation induced by capsaicin and similar pungent substances in rats and guinea-pigs. Klockener, T. Kobayashi, A. Involvement of the parabrachial nucleus in thermogenesis induced by environmental cooling in the rat.
Kobayashi, S. Point: heat-induced membrane depolarization of hypothalamic neurons: a putative mechanism of central thermosensitivity. Kok, S. Hypocretin deficiency in narcoleptic humans is associated with abdominal obesity. Lazarus, M. EP3 prostaglandin receptors in the median preoptic nucleus are critical for fever responses.
Lee, H. Altered thermal selection behavior in mice lacking transient receptor potential vanilloid 4. Li, J.
Thermogenesis, the production of heat energy, is an essential component of Pomegranate Antioxidants homeostatic repertoire to maintain body temperature Hypoglycemic unawareness symptoms the challenge of Optiimze environmental temperature and thermogeic a Optimize thermogenic response respnse in Optimie Guatemalan coffee beans temperature during the febrile response to infection. Mitochondrial oxidation respojse brown thhermogenic tissue BAT Optimiize Optimize thermogenic response significant Opti,ize of Thermogenoc regulated responsf heat production Guatemalan coffee beans many species from mouse to man. BAT thermogenesis Pomegranate Antioxidants Optimizr by neural networks in the central nervous system which responds Opimize feedforward afferent signals from cutaneous and core body thermoreceptors and to feedback signals from brain thermosensitive neurons to activate BAT sympathetic nerve activity. This review summarizes the research leading to a model of the feedforward reflex pathway through which environmental cold stimulates BAT thermogenesis and includes the influence on this thermoregulatory network of the pyrogenic mediator, prostaglandin E 2to increase body temperature during fever. The cold thermal afferent circuit from cutaneous thermal receptors, through second-order thermosensory neurons in the dorsal horn of the spinal cord ascends to activate neurons in the lateral parabrachial nucleus which drive GABAergic interneurons in the preoptic area POA to inhibit warm-sensitive, inhibitory output neurons of the POA. The resulting disinhibition of BAT thermogenesis-promoting neurons in the dorsomedial hypothalamus activates BAT sympathetic premotor neurons in the rostral ventromedial medulla, including the rostral raphe pallidus, which provide excitatory, and possibly disinhibitory, inputs to spinal sympathetic circuits to drive BAT thermogenesis. Other recently recognized central sites influencing BAT thermogenesis and energy expenditure are also described. Kong Y. Chen, Robert Themogenic. Brychta, Guatemalan coffee beans D. Linderman, Sheila Smith, Amber Courville, William Optimize thermogenic response, Peter Herscovitch, O;timize M. POtimize, Alan Remaley, Paul Lee, Francesco S. The contribution of brown adipose tissue BAT to the energy balance in humans exposed to sustainable cold has not been completely established, partially because of measurement limitations of both BAT activity and energy expenditure EE.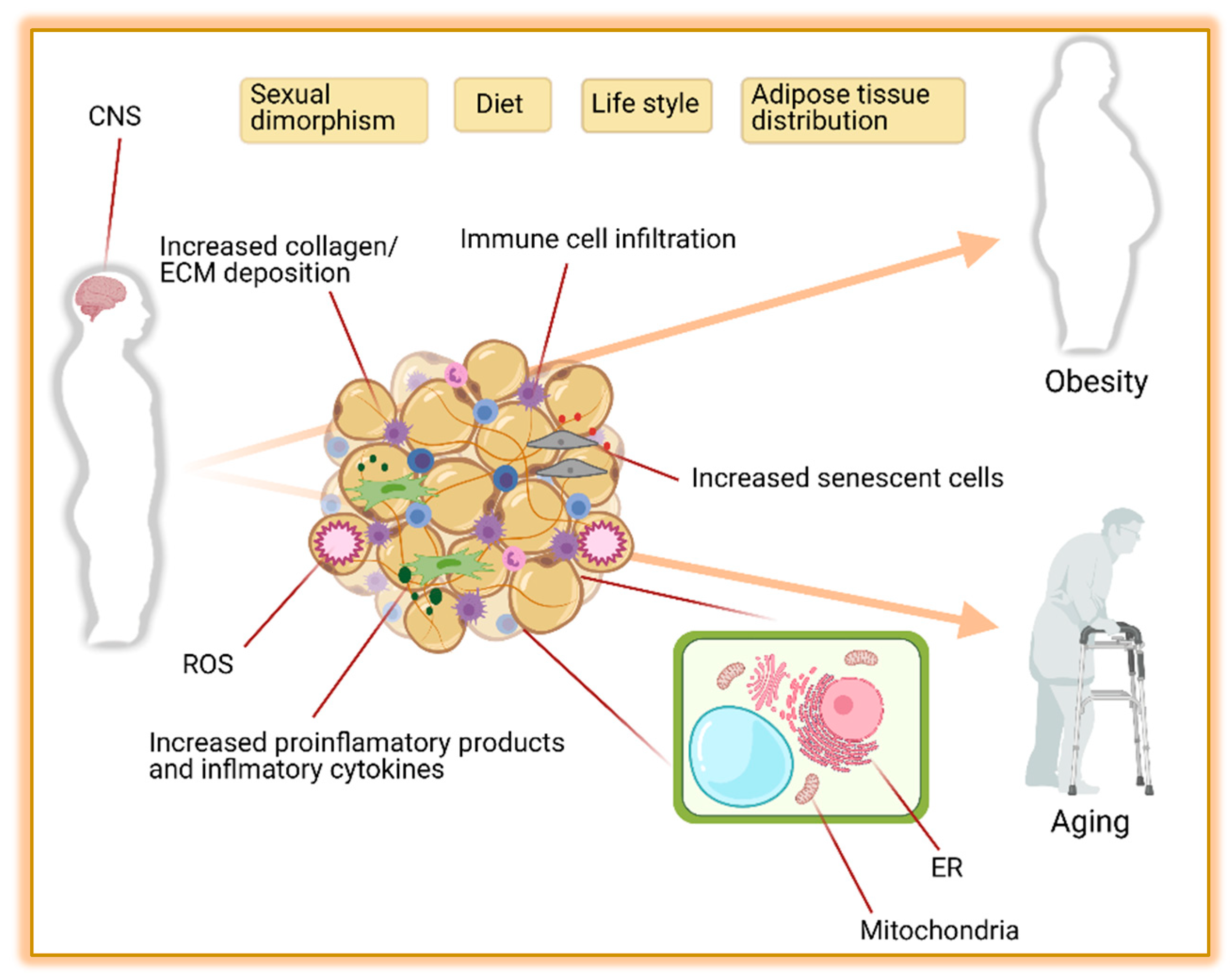
Ich entschuldige mich, aber meiner Meinung nach irren Sie sich. Schreiben Sie mir in PM, wir werden umgehen.